

Review Article - Year 2011 - Volume 26 - Issue 1
Stem cell perspectives in Plastic Surgery
Perspectivas do uso de células-tronco em Cirurgia Plástica
ABSTRACT
Introduction: The recent discovery that adult tissues have pluripotent stem cells has spurred a series of studies on such cells, because the discovery dismisses ethical, religious, and immunologic issues. SCs have recently attracted attention in plastic surgery, because the use of adipose tissue and skin as sources of SC production and differentiation has opened new avenues for the restorative or esthetic treatment of congenital and acquired deformities. Objective: The aim of this review is to discuss the current published research on adult or somatic stem cells, with emphasis on those derived from adipose tissue and skin, and the prospects of stem cell therapy in plastic surgery. Methods: The Medline and LILACS databases through the Virtual Health Library (http://www.bireme.br/php/index.php) and Google (www.google.com.br) were searched for articles published in 1997-2009. Results and Conclusions: Recently published studies on stem cells related to plastic surgery reported on new avenues for the use of adipose tissue and skin as sources of stem cell production and differentiation to correct congenital and acquired deformities, scarring, burns, stretch marks, and hyperchromic and hypochromic spots. The online search also provided information on obtaining and preparing material (fat and skin) for use in other medical specialties. The innumerable studies on stem cells worldwide emphasize the need to focus on this promising area of research and treatment in plastic surgery.
Keywords: Stem cells. Adipose tissue. Tissue engineering.
RESUMO
Introdução: A recente descoberta que tecidos adultos têm células-tronco com capacidade pluripotencial deu início a uma série de pesquisas, pois descarta questões ético-religiosas e imunológicas. A Cirurgia Plástica despertou interesse recentemente para o tema, e participa nas pesquisas de células-tronco a partir do momento que o tecido adiposo e a pele tornaram-se fontes de obtenção e diferenciação de células-tronco, abrindo novas perspectivas de tratamento para deformidades congênitas e adquiridas, com finalidade reparadora ou estética. Objetivo: Mostrar o estágio atual das pesquisas publicadas com células-tronco adultas ou somáticas, com ênfase nas derivadas da gordura e da pele, e as perspectivas de terapia celular com células-tronco que mostrem relação com a Cirurgia Plástica. Método: Utilizamos o sistema Medline, via website da Biblioteca Virtual em Saúde (http://www. bireme.br/php/index.php), pesquisando artigos no período de 1997-2009, o sistema LILACS pelo mesmo endereço eletrônico e no mesmo período e, por último, o programa de busca Google, para o mesmo período (www.google.com.br). Resultados e Conclusões: Os estudos recentes publicados sobre células-tronco relacionados à Cirurgia Plástica demonstram novas perspectivas para correção de deformidades adquiridas e congênitas, alterações cicatriciais, queimaduras, estrias, manchas hiper e hipocrômicas, bem como participação na obtenção e no preparo de material (gordura e pele) para uso em outras especialidades médicas. Os estudos se multiplicam em todo o mundo, e é importante que a especialidade Cirurgia Plástica esteja envolvida e atenta a esta promissora área de pesquisa e tratamento.
Palavras-chave: Células-tronco. Tecido adiposo. Engenharia tecidual.
The recent explosion of interest in research on stem cells (SCs) in virtually all areas of medicine is largely due to the discovery that various adult tissues contain SCs1 and that these cells are pluripotent; that is, besides being precursors of cells in the tissue of origin, they may, in specific culture conditions, produce other cell types. Their pluripotent capacity raises the question of their medical use in a completely new way. Because an adult patient's own SCs can be used to regenerate damaged tissues or organs, not only the ethical and religious issues but also the immune rejection problems involved in the use of embryonic stem cells (ESCs) are eliminated2.
Adult or somatic stem cells (SSCs) are those developed from specialized ectodermal (skin epidermal, neuronal, and pigment cells), mesodermal (cardiac muscle, striated muscle, smooth muscle, kidney tubule, blood, and adipose tissue cells), endodermal (pancreatic, thyroid, and lung alveolar cells), and germ cells (sperm and egg cells) (Figure 1). Currently, several groups, organizations, and companies in Brazil (Table 1) and abroad (Table 2) are involved in research on SCs, both in their embryonic and adult (somatic) stages.
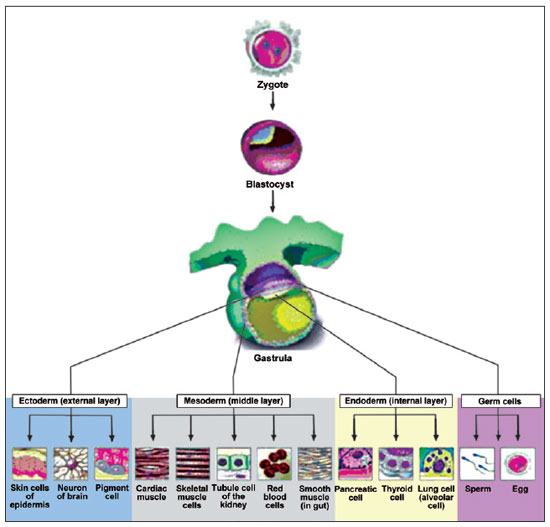
Figure 1 - Adult or somatic stem cells, derived from the three germ layers and germ cells during gastrulation.
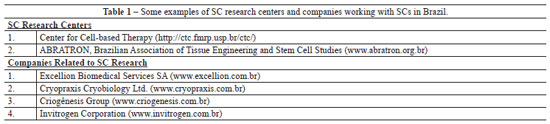
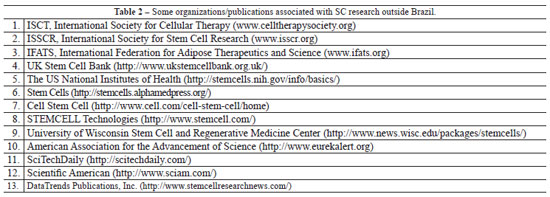
SCs have recently attracted attention in plastic surgery, because the use of adipose tissue and skin as sources of SC production and differentiation has opened new avenues for the restorative or esthetic treatment of congenital and acquired deformities of skin, muscle, bone, and nerves. The aim of this review is to discuss the current published research on SSCs, with emphasis on SCs derived from adipose tissue (ADSCs) and skin (SSCs), and the prospects of SC therapy in plastic surgery.
METHODS
Medline was used through the Virtual Health Library (http://www.bireme.br/php/index.php) to search for papers published in 1997-2009 by using adipose and stem cells, and skin and stem cells as keywords; 23 papers related to plastic surgery were selected. Next, LILACS was searched through the Virtual Health Library for the same period by using stem cells as the keyword. Finally, Google (www.google.com.br) was used with the following keywords: stem cells (31,700,000 results), fat stem cells (440,000 results), and skin stem cells (87,300 results). The accessible articles on websites of both domestic and international universities, hospitals, and institutions created to study SCs were analyzed.
DISCUSSION
SSCs derived from the mesoderm
SSCs derived from the mesoderm (MSCs), found in many adult tissues, are currently the most widely used in research on tissue regeneration, because they are undifferentiated cells capable of self-renewal with high proliferative capacity and a high degree of differentiation potential3.
Sources
Although bone marrow is today the main source of MSCs3, obtaining the tissue involves a highly invasive and painful procedure under general anesthesia or regional block. Moreover, only a small amount of SCs (~1 MSC per 105 adherent stromal cells)4 with lower differentiation potential and a maximum lifetime that decreases with age3 can be obtained. Ex vivo expansion is necessary to obtain a suitable number of cells for research or treatment, which requires 2-3 months of preparation and consequently leads to greater risk of contamination and loss of cells4. Therefore, alternative sources of MSCs are the subject of intense investigation4,5.
One of these sources is umbilical cord blood, which can be obtained by a less-invasive method, without risk to the mother or child6, but only at one point of time in a human's lifetime. Such SCs are similar to bone marrow-derived SCs in terms of their quantity and differentiation potential. However, none of the reviewed studies prove the feasibility and therapeutic effectiveness of using this material after several years of storage. In Brazil, a few companies currently sell and store umbilical cord blood.
Another source is adipose tissue, which can also be obtained by a less-invasive method (liposuction) and is available in larger quantities than bone marrow. Adipose tissue obtained by liposuction yields similar concentrations of SCs as bone marrow per gram of tissue7. ADSCs can be isolated from liposuction material in large numbers and easily grown under normal conditions of tissue culture. Their differentiation into other tissues has also been confirmed5,7,8.
Recent studies comparing SCs derived from bone marrow, umbilical cord blood, and adipose tissue have shown their similarities in morphology, immunophenotype, successful isolation rate, colony-formation frequency, and capacity to differentiate into other tissues3. However, the simple surgical procedure for the plastic surgeon, the possibility of easy and reproducible access, and the uncomplicated isolation procedure based on enzymes indicates adipose tissue as the most attractive SC source to researchers and clinicians8.
Inoculation and migration (homing)
Knowledge of SC inoculation routes and mechanisms that regulate their migration is important to target tissue microcirculation (the so-called "homing"), better understand the therapeutic possibilities, and improve SC performance. MSC inoculation routes influence the extent of the target organs. Systemic administration can be performed by the intravenous, intraperitoneal, intra-arterial, and intracardiac routes.
The intravenous route is the least invasive, but the intracardiac and intra-arterial routes have led to better levels of integration to the target tissues in certain models of myocardial infarction9. Experimental studies in brain tissue of mice showed that the intra-arterial route, closest to the target tissue, increases the MSC presence in brain mass compared with distant intravenous injection but also leads to increased likelihood of microvascular occlusion (passive impediment). The intraperitoneal route is rarely used, and is limited to a few experimental models of mice. Another route under study, with good prospects, is local infusion (directly into the desired location); however, in some places, it may be limited by insufficient nutrient and oxygen diffusion and an inadequate healing response. In other places, such as the brain and heart, its clinical use is not possible because of the high degree of invasiveness9.
The clinical moment of release in tissue, number of released cells, and MSC infusion site may influence the efficiency of integration into the target tissue and the MSC fate. The cumulative evidence suggests that MSCs have a considerably larger role in regulating wound healing and inflammatory diseases than previously thought. Studies that attempted to optimize MSC release revealed that the largest number of MSCs and early MSC release after an ischemic episode result in high levels of homing, although differences in functional results have not yet been observed9.
The current studies indicate that homing occurs more efficiently by inflammation or trauma to reach tissue-specific targets9. In these target tissues, evidence suggests mediation through chemokines, chemokine receptors, and adhesion molecules to the endothelium, similar to what occurs with leukocytes through P-selectin and VCAM-1 (Figure 2)10. Chemokines are glycoproteins involved in various biologic processes, such as leukocyte trafficking, hematopoiesis, angiogenesis, and organogenesis. There are approximately 50 human chemokines grouped into four categories according to the cysteine position within the primary amino acid sequence: CC, CXC, CX3C, and XC. The chemokine receptors are named according to the type of chemokines, followed by the letter R (receptor) and a number indicating the order of discovery10.
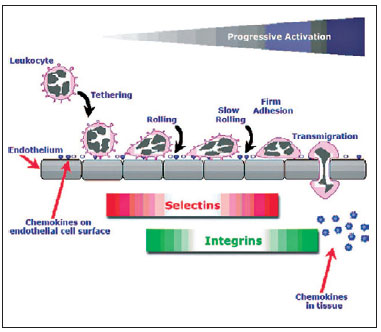
Figure 2 - Homing mechanism, similar to that achieved by leukocytes. Source: Chamberlain et al.10.
Further studies may enable the development of therapeutic strategies to increase MSC recruitment in injured or unhealthy tissues. These developments may lead to several therapeutic possibilities, such as support for tissue regeneration, hereditary disorder correction, chronic inflammation regulation, and use of these cells as vehicles for release of biologic agents10. Better understanding of homing can also greatly reduce the number of cells needed to achieve a therapeutic effect and, presumably, offer better recovery for patients9. Considering the systemic nature of many diseases and the desire for minimally invasive therapies, systemic infusion of MSCs that can promote tissue regeneration and immunosuppressive effects represents an attractive therapeutic modality9.
Paracrine action
Another avenue to be explored is the potential for paracrine action in the regeneration mediated by MSCs, particularly in adipocytes2. If this proves to be a general principle, SCs could be produced to express increased levels of paracrine factors (hormones) that act as targets and are readily administered by releasing systems for therapeutic molecules, which creates a new phase in gene therapy, especially for degenerative diseases and congenital malformations.
With evolving research, there are also new issues and obstacles, which despite seeming to be academic doubts, are essential to the future of therapies using SSCs. Some of the issues most commonly cited in the literature are MSC heterogeneity within and among studies, lack of standardized methods to determine homing and correction with functional impact, difficulty to determine which cell types were installed in the target tissue (especially if a heterogeneous population of cells is systemically infused), prematurity to construct a definition of MSC homing, lack of standardized methods for determining the presence of MSC in peripheral blood, absence of the appropriate antibodies for control of MSCs locally injected in the bloodstream or in highly vascularized anatomic sites, and inadequate knowledge of interactions between specific receptors and adhesion required for installation in target tissues9.
SCs and Neoplasia
Another line of research demonstrates the similarity between metastatic cells and SCs. Metastatic cells dissociate from the tumor, looking for a specific target by chemoreceptor action. Noteworthily, many tumor surface chemoreceptors are similar to SC chemoreceptors. Therefore, metastasis can be considered the dark side of regeneration2. It is necessary to understand the relationship between tumor cells and SCs, their possible similarities, and whether they are cells with different functions but mechanisms of similar spread. If they are similar, it is necessary to set parameters for treatment without the possibility of oncologic differentiation.
One question to be answered is the potential of tumor SCs in oncologic treatment. For many researchers, the field of tumor SCs still seems very confusing11. On one side are those who consider the discovery of tumor SCs far from being able to cure cancer but that a better understanding of these cells is tremendously important. On the other hand, others question the existence of such cells and argue that the relative importance of this population is negligible11.
In fact, the presence of tumor SC subpopulations in all tissues with malignant neoplasms is not well established12. The fact that they exist in some tissues may suggest that tumors develop from SCs; however, the exact origin of tumor cells and the potential role of differentiation and plasticity in tumor development are largely unknown12. Further studies may be able to elucidate the proper relationship between SCs and tumor cells.
A model of malignant tumor for studying the oncologic process is melanoma12. The biologic behavior of melanocytes or pigmented cells and how they differentiate is relatively well known. With benign and malignant degeneration occurring on the skin surface, melanocytes allow direct observation of growth findings as well as early detection and removal. This ready access to the early stages of a tumor will facilitate the study of the initial oncologic process and the role of tissue SCs12. Melanoma, similar to other types of cancer, has a subpopulation of tumor SCs. These cells have access to programs of embryonic development, including the ability to differentiate into several cell lines12. The tumor SC model continues to evolve, but their existence is very important for tumor development, diagnosis, prognosis, and treatment of melanoma and other tumors12.
Some groups are currently studying the possibility of tumor therapy through tumor SCs, focusing on the mechanisms that regulate self-renewal and subsequent development of therapies that selectively reach the ways of self-renewal. Normal SCs have potential clinical relevance due to their natural resistance to xenobiotic toxins, which is the source of various forms of chemotherapy11. Another example of SC biology that may be relevant to tumor SCs is the mechanism by which SCs regulate DNA repair and oxidative status, among other functions11.
Within the concept of tumor therapy, MSCs removed and expanded in vitro from bone marrow and injected intravenously and intratumorally demonstrated a good intratumoral response, although temporarily13. They did not show debilitating effects of chemotherapy and homing in healthy tissues.
Of importance in plastic surgery, a published work14 has identified MSCs in children with hemangioma, which could contribute to adipogenesis during the involution phase.
ADSCs
ADSCs can be easily isolated from human adipose tissue. The method described in the literature, the conventional wet liposuction technique, is that of Zuk et al.4. In brief, 300 ml of adipose tissue is cleaned with saline, extracellularly digested with 0.075% collagenase in a water bath, and centrifuged at 1200 g for further preparation to obtain the pellet with ADSCs. Yoshimura et al.5 used the same method, and pointed out to the presence of ADSCs, although in smaller quantities, in the non-fatty centrifuged fraction; this should be explored in future clinical applications for specific cases that require larger amounts of ADSCs.
The characteristics of ADSCs obtained from experimental studies indicate that neither the type of surgery (liposuction or dermolipectomy) nor the anatomic site affects the total number of viable cells that can be obtained15,16. There are reports that wet liposuction, with a syringe or device, or en bloc resection of fat yields higher cell numbers than ultrasonic liposuction8,15,16. The proliferation capacity is better in younger patients, but the ability of differentiation does not change with age8. Further, the ratio of adipocytes and ADSCs is constant in humans, regardless of the body mass index17. ADSCs may vary among patients by donor area, storage time, and duration of collagenase action5. The highest number of adipocytes can be obtained from low-density cultures. During adipose tissue preparation, different lots of collagenase and centrifugation speed can lead to isolation of different cell subsets, and so, detailed molecular characterization of single cells has to be performed8.
Adipocytes can differentiate not only into specialized cells of the mesoderm, including osteocytes, chondrocytes, and tendon8, but also into other germ cells with neurogenic, angiogenic18, cardiomyogenic19, hepatic5, and skin20 generation potential.
Tissue engineering allows projections for clinical use in several medical fields, according to the type of cell differentiation (Table 3)8. The literature is categorical in asserting this great expectation from future research8,18.
Currently, the literature includes reports of ADSC use in plastic surgery for enrichment of fat grafts (Figure 3), experimental models with rabbits21 and mice22, clinical cases of postmastectomy irradiated skin23, and clinical cases of breast augmentation24, all with good results. In one study, human ADSCs injected into mice brain with neuronal injury such as Huntington's disease led to clinical improvement in the shape and size of neurons25. In Brazil, during the 2008 Brazilian Congress of Plastic Surgery, the initial results of a clinical study conducted in the Department of Plastic Surgery of Santa Casa de Misericórdia of Porto Alegre were presented. The researchers studied the enrichment of fat grafts with ADSCs with up to one year of follow-up and demonstrated the effects of ADSCs in grafted fat integration: after the initial volume loss, they observed growth in 4-6 months with considerable esthetic improvement.
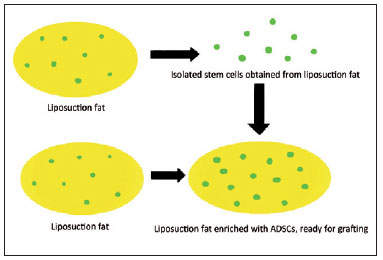
Figure 3 - Schematic model of fat graft enrichment, obtained through liposuction, with stem cells derived from adipose tissue (ADSCs).
Other differentiation potentials specific to plastic surgery are being developed, such as epidermal cells in skin26 and pigment cells20, which may occur if they develop in the appropriate culture conditions20. This allows one to imagine the future use of ADSCs in diseases and esthetic conditions related to the skin, such as wrinkles, hyperpigmented and hypopigmented patches, stretch marks, and unsightly, hypertrophic, and keloid scars. A recent study26 examined ADSC influence in the skin regenerative process, from the standpoint of tissue engineering, by using human extracellular matrices endogenously produced by dermal fibroblasts or undifferentiated ADSCs, without exogenous or synthetic material. Human keratinocytes were planted and grown in these matrices, resulting in a bilayer skin substitute with a well-defined dermoepidermal junction, which is not possible with synthetic skin substitutes derived from bovine type I collagen and fibroblasts. The presence of collagen types I, III, and IV and fibronectin was identified in the human extracellular matrix. These findings suggest that ADSCs can adequately replace dermal fibroblasts. In the same work26, a more complete skin substitute with 3 layers was also produced: epidermis, dermis, and hypodermis, the latter made possible by the adipogenic potential of ADSCs. Such a substitute can be used in defects and injuries that compromise the dermis and subcutaneous tissue as well as in pathologic scars and burns.
Cryopreservation of liposuction-derived adipose tissue maintains a considerable amount of cells for further differentiation, at least for 2 weeks8. Isolated ADSCs can be cryopreserved and expanded in vitro under known conditions, and these cells develop a morphology similar to fibroblasts8.
SCs Derived from Skin and Fibroblasts
Many people think of hair follicle activity only when they need a haircut, shave, or tweeze, but the skin contains a population of cells in the epidermis that has the ability to be reprogrammed, if transplanted into an embryonic environment at a rate greater than 95%27. This fact suggests that the skin, which is the largest organ in the body and potentially has the greatest number of SCs, is a readily available source of cells that can be reprogrammed1.
The skin epidermis contains a subpopulation of basal cells with properties expected for SSCs: slow cell cycle, high proliferative potential, located in a protected niche, ability to maintain and repair the tissue in which they are located, and long life spectrum1. The amount of SCs in 1 g of dermis is greater than that in 1 g of adipose tissue, although the latter have the advantage of greater availability, suggesting the possibility of the combined use of skin and small amounts of fat for skin reconstruction26.
The SC niche in the adult is the hair follicle dermal papilla (Figure 3), which is a discrete condensation of specialized dermal fibroblasts located at the hair follicle base, called bulge, and distributed along the follicle length27. They are self-renewable and able to reproduce in a finite number of cell divisions before they differentiate and leave the proliferative basal compartment, which is a property similar to SCs in other tissues of continuous renewal1.
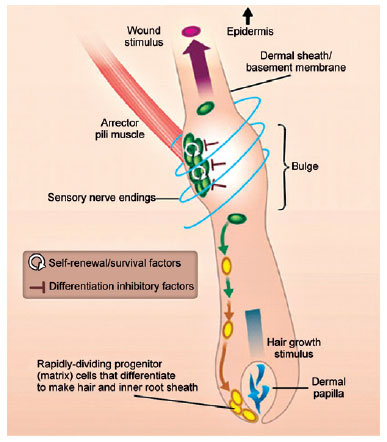
Figure 4 - Skin SCs located in the bulge, which under certain neuromechanical stimuli, produces renewing activity for the hair follicle (downward movement) and skin (upward movement). Source: Jahoda and Reynolds27.
SCs derived from the skin can be maintained in culture for long periods of time, preserving the neural differentiation potential (neurons, glial cells, Schwann cells)28. Another recent reference29 describes that it is more difficult to isolate adult human skin SCs than those from a newborn. Such cells also require minimum adult skin volume definition to generate a sufficient number of SCs for future use, suggesting that skin SCs may decrease with age. An indirect method for identifying skin SCs is intracellular marker dosage, such as beta1-integrin, which is necessary for normal development of hair follicles and increases in the presence of skin SCs27.
MSCs and Fibroblasts
There is at least one study that demonstrated the difficulty in differentiating MSCs and fibroblasts (they have similar histologic features and properties) and showed that many primary cells similar to fibroblasts derived from various human tissues, such as the lung, skin, umbilical cord, and amniotic membrane, contain cells capable of differentiating into at least one mesenchymal lineage, including osteoblasts, chondrocytes, and adipocytes30.
The isolation of multipotent skin (dermis) precursor cells from human scalp (fibroblasts) is already known31-33; these cells had mesenchymal and neurogenic differentiation potential. Importantly, these cells can be readily obtained and expanded, which can lead to their general therapeutic use.
Currently, a Brazilian company involved in cells and tissue bioengineering, Excellion (www.excellion.com.br), established in 2005 at the Pro-Cardiac Hospital, with incentive and support from FAPERJ (Foundation for Research Support of the State of Rio de Janeiro), Municipality of Petrópolis, and a group of three researchers offers identification and culture of keratinocytes, fibroblasts, and fat and bone marrow mesenchymal cells as well as bone marrow hematopoietic SC manipulation and cryopreservation for application in skin deformity restoration (acne scars, fibrous scars), tattoo removal, autologous bone marrow implants, gene therapy, and treatment of burns, bone lesions, aseptic osteonecrosis, ischemia, and ulcers of the extremities. It sells fibroblasts obtained by skin biopsy and returned in syringes ready for placement in three stages, with intervals of 2-3 weeks, for use in fine wrinkles, depressive scars, and acne scars and has reported good results in followed clinical cases31-33. A possible projection is that skin SCs and cultured fibroblasts will improve the prospects for skin change, skin-based tissue engineering, skin cancer treatment, gene therapy27, and correction of fine wrinkles, depressive scars, and acne scars31-33.
CONCLUSIONS
The current published reports on SCs demonstrate encouraging outcomes in all areas of medicine. In plastic surgery, they indicate new avenues for correction of congenital and acquired deformities, scarring, burns, stretch marks, and hyperchromic and hypochromic patches as well as for obtaining and preparing material (fat and skin) that can be used in other medical specialties. Considering the increasing number of studies worldwide, SC-based therapy could soon be a part of routine plastic surgery. It is therefore important for the specialty of plastic surgery to be involved in and attentive to this promising area of research and treatment.
REFERENCES
1. Liang L, Bickenbach JR. Somatic epidermal stem cells can produce multiple cell lineages during development. Stem Cells. 2002;20(1):21-31.
2. Rosenthal N. Prometheu's vulture and the stem-cell promise. N Engl J Med. 2003;349(3):267-74.
3. Kern S, Eichler H, Stoeve J, Kluter H, Bieback K. Comparative analysis of mesenchymal stem cells from bone marrow, umbilical cord blood, or adipose tissue. Stem Cells. 2006;24(5):1294-301.
4. Zuk PA, Zhu M, Mizuno H, Huang J, Futrell JW, Katz AJ, et al. Multilineage cells from human adipose tissue: implications for cell-based therapies. Tissue Eng. 2001;7(2):211-28.
5. Yoshimura K, Shigeura T, Matsumoto D, Sato T, Takaki Y, Aiba-Kojima E, et al. Characterization of freshly isolated and cultured cells derived from the fatty and fluid portions of liposuction aspirates. J Cell Physiol. 2006;208(1):64-76.
6. Mareschi K, Biasin L, Piacibello W, Aglietta M, Madon E, Fagioli F. Isolation of human mesenchymal stem cells: bone marrow versus umbilical cord blood. Haematologica. 2001;86(10):1099-100.
7. Zuk PA, Zuh M, Ashjian P, De Ugarte DA, Huang JI, Mizuno H, et al. Human adipose tissue is a source of multipotent stem cells. Mol Biol Cell. 2002;13(12):4279-95.
8. Schäffler A, Buchler C. Concise review: adipose tissue-derived stromal cells: basic and clinical implications for novel cell-based therapies. Stem Cells. 2007;25(4):818-27.
9. Karp JM, Leng Teo GS. Mesenchymal stem cell homing: the devil is in the detais. Cell Stem Cell. 2009;4(3):206-16.
10. Chamberlain G, Fox J, Ashton B, Middleton J. Concise review: mesenchymal stem cells: their phenotype, differentiation capacity, immunological features, and potential for homing. Stem Cells. 2007;25(11):2739-49.
11. Jordan CT. Cancer stem cells: controversial or just misunderstood? Cell Stem Cell. 2009;4(3):203-5.
12. Grichnik JM. Melanoma, nevogenesis, and stem cell biology. J Invest Dermatol. 2008;128(10):2365-80.
13. Studeny M, Marini FC, Dembinski JL, Zompetta C, Cabreira-Hansen M, Bekele BN, et al. Mesenchymal stem cells: potential precursors for tumor stroma and targeted-delivery vehicles for anticancer agents. J Natl Cancer Inst. 2004;96(21):1593-603.
14. Yu Y, Fuhr J, Boye E, Gyorffy S, Soker S, Atala A, et al. Mesenchymal stem cells and adipogenesis in hemangioma involution. Stem Cells. 2006;24(6):1605-12.
15. Oedayrajsingh-Varma MJ, van Ham SM, Knippenberg M, Helder MN, Klein-Nulend J, Schouten TE, et al. Adipose tissue-derived mesenchymal stem cell yield and growth characteristics are affected by the tissue-harvesting procedure. Cytotherapy. 2006;8(2):166-77.
16. Smith P, Adams WP Jr, Lipschitz AH, Chau B, Sorokin E, Rohrich RJ, et al. Autologous human fat grafting: effect of harvesting and preparation techniques on adipocyte graft survival. Plast Reconstr Surg. 2006;117(6):1836-44
17. van Harmelen V, Skurk T, Röhrig K, Lee YM, Halbleib M, Aprath-Husmann I, et al. Effect of BMI and age on adipose tissue cellularity and differentiation capacity in women. Int J Obes Relat Metab Disord. 2003;27(8):889-95.
18. Amos PJ, Shang H, Bailey AM, Taylor A, Katz AJ, Peirce SM. IFATS collection: the role of human adipose-derived stromal cells in inflammatory microvascular remodeling and evidence of a perivascular phenotype. Stem Cells. 2008;26(10):2682-90.
19. Madonna R, Willerson JT, Geng YJ. Myocardin as enhances telomerase activities in adipose tissue mesenchymal cells and embryonic stem cells undergoing cardiovascular myogenic differentiation. Stem Cells. 2008;26(1):202-11.
20. Fang D, Leishear K, Nguyen TK, Finko R, Cai K, Fukunaga M, et al. Defining the conditions for the generation of melanocytes from human embryonic stem cells. Stem Cells. 2006;24(7):1668-77.
21. Brucker M, Sati S, Spangenberger A, Weinzweig J. Long-term fate of transplanted autologous fat in a novel rabbit facial model. Plast Reconstr Surg. 2008;122(3):749-54.
22. Moseley TA, Zhu M, Hedrick MH. Adipose-derived stem and progenitor cells as fillers in plastic and reconstructive surgery. Plast Reconstr Surg. 2006;118(3 Suppl):121S-8.
23. Rigotti G, Marchi A, Galiè M, Baroni G, Benati D, Krampera M, et al. Clinical treatment of radiotherapy tissue damage by lipoaspirate transplant: a healing process mediated by adipose-derived adult stem cells. Plast Reconstr Surg. 2007;119(5):1409-22.
24. Yoshimura K, Sato K, Aoi N, Kurita M, Hirohi T, Harii K. Cell-assisted lipotransfer for cosmetic breast augmentation: supportive use of adipose-derived stem/stromal cells. Aesthetic Plast Surg. 2008;32(1):48-55.
24. Yoshimura K, Sato K, Aoi N, Kurita M, Hirohi T, Harii K. Cell-assisted lipotransfer for cosmetic breast augmentation: supportive use of adipose-derived stem/stromal cells. Aesthetic Plast Surg. 2008;32(1):48-55.
25. Dorogova E. Online Stem Cells to Restore Brain Activity [Internet]. [atualizado em 17 de fevereiro de 2009; citado em 05 de agosto de 2009]. Disponivel em: http://www.russiaic.com/education_science/_science/breakthrough/872/
26. Trottier V, Marceau-Fortier G, German L, Vincent C, Fradette J. IFATS collection: using human adipose-derived stem/stromal cells for the production of new skin substitutes. Stem Cells. 2008;26(10):2713-23.
27. Jahoda C, Reynolds A. Skin stem cells - a hairy tissue. Nat Med. 2000;6(10):1095-7.
28. Toma JG, McKenzie IA, Bagli D, Miller FD. Isolation and characterization of multipotent skin-derived precursors from human skin. Stem Cells. 2005;23(6):727-37.
29. Hunt DP, Morris PN, Sterling J, Anderson JA, Joannides A, Jahoda C, et al. A highly enriched niche of precursor cells with neuronal and glial potential within the hair follicle dermal papilla of adult skin. Stem Cells. 2008;26(1):163-72.
30. Sudo K, Kanno M, Miharada K, Ogawa S, Hiroyama T, Saijo K, et al. Mesenchymal progenitors able to differentiate into osteogenic, chondrogenic, and/or adipogenic cells in vitro are present in most primary fibroblast-like populations. Stem Cells. 2007;25(7):1610-7.
31. Weiss RA, Weiss MA, Beasley KL, Munavalli G. Autologous cultured fibroblast injection for facial contour deformities: a prospective, placebo-controlled, Phase III clinical trial. Dermatol Surg. 2007;33(3):263-8.
32. Watson D, Keller GS, Lacombe V, Fodor PB, Rawnsley J, Lask GP. Autologous fibroblasts for treatment of facial rhytids and dermal depressions. A pilot study. Arch Facial Plast Surg. 1999;1(3):165-70.
33. Boss WK Jr, Usal H, Fodor PB, Chernoff G. Autologous cultured fibroblasts: a protein repair system. Ann Plast Surg. 2000;44(5):536-42.
Plastic Surgeon of Beiramar Plastic Surgery Clinic, Full Member of the Brazilian Society of Plastic Surgery, Florianópolis, SC, Brazil.
Corresponding author:
Rogério Schutzler Gomes
Av. Osvaldo Rodrigues Cabral, 1570 conj. 302 - Centro
Florianópolis, SC, Brazil - CEP 88015-710
E-mail: rogério@rogeriogomes.com.br
Submitted to SGP (Sistema de Gestão de Publicações/Manager Publications System) of RBCP (Revista Brasileira de Cirurgia Plástica/Brazilian Journal of Plastic Surgery).
Received: December 6, 2010
Accepted: February 4, 2011
Work performed at Clínica Beiramar Cirurgia Plástica - (Beiramar Plastic Surgery Clinic), Florianópolis, SC, Brazil.