

Original Article - Year 2013 - Volume 28 -
Does hyperbaric oxygenation have a protective effect on random skin flaps? An immunohistochemical study of cellular apoptosis and vascular endothelial growth factor
Existe efeito protetor da oxigenação hiperbárica em retalhos cutâneos randômicos? Um estudo imuno-histoquímico de apoptose celular e fator de crescimento vascular endotelial
ABSTRACT
BACKGROUND: Hyperbaric oxygenation (HBO) therapy aims to increase oxygen tissue pressure by at least 10 times and decrease the adverse effects of ischemia. The aim of this study was to investigate the effect of HBO on the immunohistochemical expressions of caspase 3 and vascular endothelial growth factor (VEGF) in random flaps in rats.
METHODS: Thirty-two male Wistar rats were randomly divided into 4 groups: the sham group (GS), the N-acetylcysteine group (GNAC), the HBO group, and the HBO + N-acetylcysteine group. A rectangular skin flap (2 × 8 cm) was dissected from the dorsal muscle layer, and a cranial pedicle was preserved. A polyethylene film was placed on the muscle layer, and the flap was fixed in the original site. On the eighth day, biopsy samples (2 × 1 cm) of the entire thickness of the proximal, medial, and cranial areas as well as of the site outside the flap, which was used as a control, were collected.
RESULTS: VEGF expression in the skin layers and vessels was not significantly different between the groups. The number of apoptotic cells was significantly increased in the area of the flap in all groups. The highest increase was noted in the GS and GNAC groups. HBO significantly reduced the number of caspase 3-positive cells in the skin layers and vessels of the 3 areas.
CONCLUSIONS: HBO was associated with decreased apoptosis. VEGF expression in the skin layers and vessels did not differ significantly. The results suggest that oxygen diffusion through the interstitial space was the determining factor for the positive effect of HBO on the decrease in apoptosis.
Keywords: Hyperbaric oxygenation. Caspase 3. Endothelial growth factors. Surgical flaps.
RESUMO
INTRODUÇÃO: A oxigenação hiperbárica (OHB) tem por objetivo aumentar em pelo menos 10 vezes a pressão tecidual de oxigênio, diminuindo os efeitos deletérios da isquemia. O objetivo deste estudo é investigar o papel da oxigenação hiperbárica na expressão imuno-histoquímica da caspase 3 e do fator de crescimento endotelial vascular (VEGF) em retalhos randômicos em ratos.
MÉTODO: Trinta e dois ratos Wistar machos foram divididos aleatoriamente em quatro grupos: grupo sham (GS), grupo N-acetilcisteína (GNAC), grupo OHB (GOHB) e grupo OHB + N-acetilcisteína (GHN). Um retalho de pele retangular (2 cm x 8 cm) foi dissecado a partir da camada muscular dorsal, preservando um pedículo cranial. Uma lâmina de polietileno foi colocada sobre a camada muscular e fixou-se o retalho no local original. No 8º dia, foram coletadas biópsias (2 cm x 1 cm) de espessura total das áreas proximal, média e cranial e de um local fora do retalho, que serviu como área de controle.
RESULTADOS: A expressão de VEGF nas camadas da pele e nos vasos não apresentou diferenças significativas entre os grupos. As células apoptóticas estavam significativamente aumentadas na área central do retalho em todos os grupos. O maior aumento ocorreu nos grupos GS e GNAC. A OHB diminuiu significativamente o número de células caspase 3 positivas nas camadas da pele e nos vasos das três áreas.
CONCLUSÕES: A OHB foi associada a expressão reduzida de apoptose. A expressão de VEGF em camadas da pele e vasos não demonstrou diferença significativa. Os resultados sugerem que a difusão do oxigênio através do espaço intersticial foi o fator determinante para os resultados mais favoráveis da OHB na diminuição da expressão de apoptose.
Palavras-chave: Oxigenação hiperbárica. Caspase 3. Fatores de crescimento endotelial. Retalhos cirúrgicos.
Hyperbaric oxygenation (HBO) is a form of therapy in which 100% medicinal oxygen is supplied under pressure to increase the oxygen pressure in tissues by at least 10 times and reduce the adverse effects of ischemia. This type of treatment has been used since 1968, when its clinical indications were defined. In plastic surgery, HBO is indicated for the treatment of patients with severe burns and for ischemic flaps because it provides hyperoxygenation to tissues, activates fibroblasts, modulates inflammatory response, accelerates growth factors, and has antibacterial activity1-5.
McFarlane et al.6 developed an experimental model to assess the viability of random flaps in rats, which had been used in various previous studies. The authors observed that the biochemical and morphological changes were directly related to the distance from the extremity of the flap and the size of its pedicle7-9.
Random flaps are exposed to tissue damage resulting from postischemic reperfusion, in which reintroduction of molecular oxygen facilitates the production of critical levels of energy, increasing the inflammatory response and causing toxicity in endothelial cells. NAcetylcysteine (NAC) is a prodrug that regulates the glutathione levels in the body when they are reduced in the presence of toxic oxygen radicals (TORs). Glutathione is one of the most potent free radical scavengers, and its cellular levels are quickly depleted during damage caused by the ischemia-reperfusion phenomenon6,10-12.
The aim of this study was to assess the effect of HBO, NAC, and their association on random flaps in rats by using an apoptosis marker (cleaved caspase 3) and an endothelial vascular growth marker (VEGF).
METHODS
Ethical Considerations
The experimental protocol (0321/06) was approved by the ethics committee of the Federal University of São Paulo. All procedures were conducted in strict accordance with the existing regulations on animal experimentation of the Colégio Brasileiro de Experimentação Animal.
Samples and Groups
Thirty-two male Wistar rats weighing 280 to 300 g were kept in individual cages in a sound-attenuated room maintained at 25ºC under artificial lighting and given food and water ad libitum. The animals were randomly divided into 4 groups as follows:
Anesthesia ProcedureGS - the sham group, including 8 animals; GNAC - the NAC group, including 8 animals; GHBO - the HBO group, including 8 animals; GHN - the HBO + NAC group, including 8 animals.
After 6 h of solid food fasting and 4 h of liquid fasting, the animals received 5 mg/kg of 0.2% acepromazine, intramuscular. Ten minutes later, the animals received a combination of 50 mg/kg-1 of ketamine hydrochloride and 10 mg/kg-1 xylazine hydrochloride, intramuscular.
Surgical Procedure
Under general anesthesia, trichotomy of dorsal regions was performed, and the animals were then fixed in the prone position. After demarcation of a rectangular patch (2 cm × 8 cm) of upper base, incision with a scalpel (blade No. 15) was performed, then the flap was dissected, including epidermis and dermis (Figure 1). A polyethylene film was interposed between the flap and the muscle layer, covering the entire area of the wound and acting as a barrier between the skin and muscles (Figure 2). Then, flap synthesis with polyamide thread 3.0 was performed, fixing the flap in its original position.
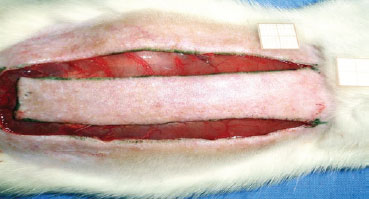
Figure 1 - Standard random skin flap (2 × 8 cm) by McFarlane et al.6 on the seventh cervical vertebra.
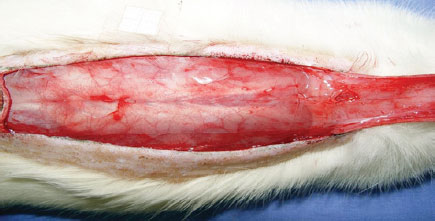
Figure 2 - Polyethylene film placed on the muscle layer, covering the entire area of the wound and acting as a barrier between the skin and the muscle.
Administration Procedures
Animals in the GNAC and GHN groups were intraperitoneally injected with 300 mg/kg of NAC (Fluimucil®; Zambon Laboratório Farmacêutico Ltda., São Paulo, SP, Brazil) after flap elevation and then every 24 hours for 7 days.
The animals in the GS and GHBO groups were intraperitoneally injected with 1 ml of distilled water (Isofarma, São Paulo, SP, Brazil) after flap elevation and then every 24 hours for 7 days.
HBO Procedures
A hyperbaric chamber for several animals was used. Before pressurization, the inside of the chamber was cleaned with 100% medicinal oxygen for 5 minutes. The chamber was then pressurized until a pressure of 2.4 atmosphere absolute (ATA). The oxygen concentration was monitored using a calibrated oximeter. All animals in the GHBO and GHN groups were exposed to 100% of oxygen at a pressure of 2.4 ATA for 2 hours (once/day), starting 15 minutes after the surgery and then every 24 hours for 7 consecutive days.
Treatment
The animals were randomly divided into the following groups:
Sample Collectionthe GS group (n = 8), received distilled water intraperitoneally 15 minutes after flap elevation and then for 7 consecutive days; the GNAC group (n = 8), received 300 mg/kg-1 NAC intraperitoneally after flap elevation and then for 7 consecutive days; the GHBO group (n = 8), received 100% oxygen at 2.4 ATA in a hyperbaric environment for 7 consecutive days for 2 hours; the GHN group (n = 8), received a combination of oxygen in a hyperbaric environment and NAC intraperitoneally for 7 consecutive days.
On the eighth day of the experiment, the rats were once again anesthetized for collection of incisional biopsy samples (2 × 1 cm), which included the entire thickness of the proximal, medial, and distal areas of the flap. As a control, another biopsy sample was collected from outside the area of the flap (Figure 3).
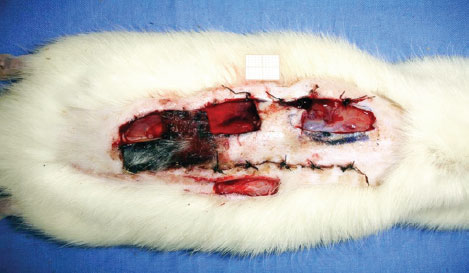
Figure 3 - Areas from where biopsy samples were collected.
Euthanasia
Under anesthesia, the animals were euthanized in a carbon dioxide chamber after sample collection.
Histological Procedure
The samples were fixed in 10% formalin, embedded in paraffin, and immunohistochemically stained to examine the expression of the apoptosis marker and VEGF.
Immunohistochemical Staining for VEGF
An anti-VEGF marker (Sigma Chemical Company, St. Louis, USA) was used to detect VEGF expression. All procedures were performed according to the manufacturer instructions.
Immunohistochemical Staining for the Apoptosis Marker
The avidin-biotin standard method was used to detect apoptotic cells using a detection kit containing anti-caspase 3 primary antibody (Lexington, USA). After incubation, the specimens were treated with rabbit immunoglobulin/biotinylated antibody (at 1:600 dilution; Dako Ltd., Ely, UK) for 30 min. All procedures were performed according to the manufacturer instructions.
VEGF Expression
Medium-power magnification ('100 and '200) was used to examine VEGF expression in the biopsy samples from the epidermis, dermis, vessels, and submuscular area. VEGF expression was classified according to the intensity of the color of the cytoplasm (from 0 to 3):
Apoptosis Marker Expression0 - no expression (negative reaction); 1 - low expression (weak brown reaction); 2 - moderate expression (moderate brown reaction); 3 - high expression (strong brown reaction).
Images were obtained using a high-resolution camera with Axiomcam software (Mission-oriented Resilient Clouds; Zeiss®) and analyzed using an optical microscope (Carl Zeiss Axiolab®). Axiovision Rel 4.2 software (Zeiss®) was used to calculate the ratio of brown cells (i.e., apoptotic cells) to non-brown cells (i.e., non-apoptotic cells). Apoptotic cells were counted in 10 fields per slide in all the samples for all the groups.
Statistical Analysis
All procedures for histological and immunohistochemical analyses were performed with the examiner blinded to the identity of the groups to which the morphological samples belonged.
For the analysis of the immunohistochemical results, nonparametric tests were applied, taking into consideration the variables under study and the sample size. Two variables were analyzed, a quantitative variable (cleaved caspase 3) and a semiquantitative variable (VEGF).
Cleaved caspase 3 levels were expressed as mean and standard deviation values. VEGF expression was presented as a score, and mean and standard deviation values were obtained for each group. Analysis of variance was performed to determine within-group differences, and the Bonferroni test was used to compare the groups.
The probability of rejecting the null hypothesis was set at 0.05 or 5%, and a P value of <0.05 was considered statistically significant (with a 95% confidence interval).
RESULTS
Regarding VEGF expression, all the biopsy samples collected from the distal third exhibited necrosis. Data on VEGF expression in the epidermis, dermis, panniculus carnosus, and vessels showed that HBO did not promote any significant change in both the GS and the GNAC groups (Table 1). Moreover, no significant difference was observed in the GHN group. These findings suggest that OHB, NAC, or the combination of both did not promote changes in VEGF expression in the tissues.
HBO caused a significant reduction in apoptotic cells in all the biopsy samples obtained from the proximal and medial thirds. The combination of HBO and NAC, in addition to not having a protective effect, exerted a negative effect (Figures 4 and 5).
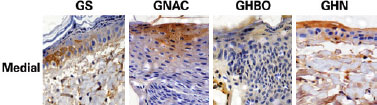
Figure 4 - Photomicrographs of the GS, GNAC, GHBO, and GHN groups, showing caspase 3 expression (brown color) in the hypodermis samples collected from the medial part of the flap (original magnification '200). GHN = GNAC + GHBO group; GNAC = N-acetylcysteine group; GHBO = hyperbaric oxygenation group; GS = sham group.
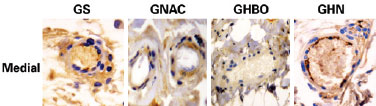
Figure 5 - Photomicrographs of the GS, GNAC, GHBO, and GHN groups, showing caspase 3 expression (brown color) in the vessels collected from the medial part of the flap (original magnification '400). GHN = GNAC + GHBO group; GNAC = N-acetylcysteine group; GHBO = hyperbaric oxygenation group; GS = sham group.
Statistical analysis of the biopsy data for the proximal, medial, and distal thirds of the flaps showed that sham (GS) group had greatest number of apoptotic cells followed by the NAC and GHN groups. The GHBO group presented fewer cells apoptosis in all strata of the epithelium under study (epidermis, dermis, submuscular area, and vessels), as shown in Table 2.
DISCUSSION
The mean oxygen tension in ischemic flaps is only 30 mmHg. Hypoxia occurs as a normal stimulus for wound healing; however, this situation can deteriorate if the local conditions of poor irrigation persist, thus impairing wound healing and leading to tissue necrosis13.
During the ischemic period, random flaps are subjected to hypoxia and hypoglycemia. These conditions lead to cellular dysfunction and cell death. When blood flow is restored, a second event known as flow/no-reflow occurs; this phenomenon is the result of the accumulation (plug) of polymorphonuclear cells that adhere to the endothelium and are responsible for the production of TORs, which are associated with the second wave of cellular damage1,14.
TORs are responsible for oxidative damage that leads to mitochondrial changes; adenosine triphosphate depletion; increase in intracellular calcium levels; and protease, phosphatase, lipase, and nuclease activation, which results in the loss of cellular integrity14.
The phenomenon of ischemia/reperfusion is mediated by TORs. These molecules are highly unstable and extremely toxic to most biological processes by means of their effects of lipid peroxidation of cell membranes, formation of free radicals in a self-propagating cycle, and cell death due to necrosis or apoptosis5.
Studies support the hypothesis that oxygen functions as a signaling molecule for a series of events that promote wound healing. The levels of hypoxia-inducible factor alpha (HIF-α) are decreased in HBO-treated tissues. HIF-α is an important regulator of genetic expression involved in the regulation of tissue oxygenation, and its levels increase in the presence of hypoxia. The level of the protein BNip3 (a pro-apoptotic protein located in the mitochondria) increases greatly after the 10th day in ischemic tissues. High levels of oxygen induced by HBO decrease and attenuate the expression of the protein BNip3, thus modulating apoptosis despite the persistent high level of lactic acid1,4,5,15.
Therefore, HBO increases antiapoptotic activity and decreases pro-apoptotic activity; there is a subsequent reduction in the release of pro-apoptotic molecules by mitochondria and attenuation of apoptosis. The molecular basis of the protective effect of HBO on ischemic wound healing is attributable to HIF-1α modulation. Wounds treated with HBO exhibit reduced levels of HIF-1α and a consequent reduction in expression of the HIF-1α gene, as well as reduced apoptosis and inflammation1,4,5.
The process of angiogenesis begins between the first and third days after ischemia and is modulated by growth factors that are present in endothelial cells and in the extracellular matrix. VEGF is the most important stimulator of angiogenesis and its expression is associated with the initiation of vascular neoformation. In addition, it is a reliable marker for monitoring the process of vascular neoformation15-18.
Caspase 3 is an apoptosis-effector protein. It converts cytoplasmic DNAse into an active form. During cell death programming, caspase 3 induces early activation of endonucleases, causes DNA fragmentation, and impairs enzymatic processes that are vital to cells. Caspase 3 has been used in previous studies on ischemic skin flaps5,19.
Many mechanisms are involved in tissue healing; however, most studies show that the presence of growth factors is associated with the production of energy derived from glycolysis and the Krebs cycle.
HBO increases oxygen tissue pressure by up to 10 times by supplying oxygen dissolved in plasma, according to the laws of Henry and Boyle; in addition, it acts directly on neutrophils and inflammatory mediators responsible for tissue damage.
Humans have a complex antioxidant system. Glutathione is depleted during antioxidation. NAC is a prodrug that replenishes the levels of glutathione when these are depleted during the oxidative process and has been used in many experimental studies because of its low toxicity and protective effect on random flaps.
Data regarding VEGF expression in the epidermis, dermis, panniculus carnosus, and vessels indicate that HBO did not promote any significant change (Table 2). The proposed mechanism to explain the absence of increased VEGF production is that the partial pressure of oxygen does not return to the pre-ischemic levels between hyperoxygenation sessions; therefore, there is no stimulus for VEGF production5.
Because VEGF expression is upregulated under ischemic conditions, we did not expect VEGF expression to be upregulated in the groups treated with HBO (GHBO and GHN), in which higher amounts of oxygen were supplied. However, a significant increase should have been observed in the GS group. The most plausible hypothesis to explain the results of this study is the significant reduction in metabolic activity and protein synthesis caused by ischemia, which inhibits VEGF expression20.
Another hypothesis that may explain the results of this study was previously put forward by other authors, who observed significant decreases in metabolic activity and protein synthesis, including the synthesis of VEGF, under ischemic conditions20.
The high concentrations of NAC used in the GNAC group might have been responsible for the inhibition of VEGF production. Previous studies have shown that high concentrations of NAC inhibit angiogenesis and the wound healing response through an oxidant/antioxidant balance that has not yet been clarified11. However, in this study, VEGF expression was not altered in any of the groups, and all groups had a profile similar to the GS group.
The model used in this study, in which a polyethylene film was applied, might have been responsible for the absence of VEGF expression in the groups treated with NAC or HBO. Because there was no difference between these groups and the control group, we suggest that the stimulus for angiogenesis was dependent on the bed on which the flap was placed after its confection. The growth of new vessels might have been delayed because there was no contact with the bed.
Data regarding the mean number of apoptotic cells in the biopsied strata showed that, in the external part of the random flap, that is, where there was no ischemia, HBO (0.4 ± 0.5) promoted a greater decrease in caspase 3 expression compared with the GS (0.8 ± 0.9), GNAC (1.3 ± 0.5), and GHN (0.8 ± 0.7) groups. These findings allowed us to infer that HBO had a protective effect in the normal tissues by decreasing caspase 3 expression and that hyperoxidation tended to have a protective effect even in the tissues that were not exposed to ischemia; surgical trauma functions as a form of preconditioning.
The overall assessment of apoptosis in the various strata suggests that HBO has a protective effect on the medial and proximal portions of the flaps. HBO group presented reduced caspase 3 expression in the external skin of the flap, indicating that the effect of hyperoxygenation on healthy tissues was similar to that in tissues that only received the surgical stimulus or were treated with NAC. The effect of the use of NAC was not different from that in the control group, and the use of HBO did not produce a synergistic effect, which implies that the use of NAC as an antioxidant did not have a protective effect.
The combination of NAC with HBO yielded worse results than the isolated use of HBO. NAC concentrations greater than 20 mmol/L may reverse the antioxidant effect through pH inversion, cytochrome C activation, and mitochondrial pore opening, and thus lead to cell death11.
The results of this study suggest a clear reduction in apoptosis in the group treated with HBO. The most plausible hypothesis for the protective effect of HBO on cellular apoptosis is the abundant supply of oxygen to the tissues during the initial stage of ischemia, which attenuates the inflammatory response and reduces lipid peroxidation4-6.
CONCLUSIONS
Considering the positive effect of HBO on the reduction of cellular apoptosis, we suggest that this is dependent on the time of exposure or the number of exposures to hyperbaric oxygen. The rationale for using HBO, previously published results, and the results of this study suggest that more prolonged or frequent daily sessions may result in decreased apoptosis. These conclusions still need to be confirmed in further studies in this area.
HBO, NAC, or the combination of both did not affect VEGF levels. This implied that the flap was exclusively dependent on the vascular pedicle when a polyethylene film was applied. HBO and NAC were not effective in increasing VEGF expression, demonstrating the absence of a stimulus for vascular neogenesis during the period under study.
REFERENCES
1. Zamboni WA, Roth AC, Russell RC, Smoot EC. The effect of hyperbaric oxygen on reperfusion of ischemic axial skin flaps: a laser Doppler analysis. Ann Plast Surg. 1992;28(4):339-41.
2. Buras J. Basic mechanisms of hyperbaric oxygen in the treatment of ischemic-reperfusion injury. Int Anesthesiol Clin. 2000;38(1):91-109.
3. Kranke P, Bennett M, Roeckl-Wiedmann I, Debus S. Hyperbaric oxygen therapy for chronic wounds. Cochrane Database Syst Rev. 2004; (2):CD004123.
4. Bertoletto PR, Fagundes DJ, Simões MJ, Oshima CT, Montero EFS, Simões RS, et al. Effects of hyperbaric oxygen therapy on the rat intestinal mucosa apoptosis caused by ischemia-reperfusion injury. Microsurgery. 2007;27(4):224-7.
5. Vidigal J, Fagundes DJ, Simões MJ, Oshima CT, Odashiro AN, Simões RS, et al. Effect of different periods of hyperbaric oxygen on ischemia-reperfusion injury of rat skeletal muscle. Microsurgery. 2007;27(4):252-7.
6. McFarlane RM, Deyoung G, Henry RA. The design of a pedicle flap in the rat to study necrosis and its prevention. Plast Reconstr Surg. 1965;35:177-82.
7. Zhang Q, Chang Q, Cox RA, Gong X, Gould LJ. Hyperbaric oxygen attenuates apoptosis and decreases inflammation in an ischemic wound model. J Invest Dermatol. 2008;128(8):2102-12.
8. Friedman HI, Fitzmaurice M, Lefaivre JF, Vecchiolla T, Clark D. An evidence-based appraisal of the use of hyperbaric oxygen on flaps and grafts. Plast Reconstr Surg. 2006;117(7 Suppl):175S-90S.
9. Zhang T, Gong W, Li Z, Yang S, Zhang K, Yin D, et al. Efficacy of hyperbaric oxygen on survival of random pattern skin flap in diabetic rats. Undersea Hyperb Med. 2007;34(5):335-9.
10. Rocha FP, Fagundes DJ, Rivoire HC, Rech FV, Almeida MW, Pires JA. Immunohistochemical expression of apoptosis and VEGF expression on random skin flaps in rats treated with hyperbaric oxygen and N-acetylcysteine. Undersea Hyperb Med. 2011;38(3):167-74.
11. Kunnavatana SS, Quan SY, Koch RJ. Combined effect of hyperbaric oxygen and N-acetylcysteine on fibroblast proliferation. Arch Otolaryngol Head Neck Surg. 2005;131(9):809-14.
12. Rocha FP, Fagundes DJ, Pires JA, Rocha FS. Effects of hyperbaric oxygen and N-acetylcysteine on the survival of random pattern skin flaps in rats. Indian J Plast Surg. 2012;45(3):453-8.
13. Weiss SJ. Oxygen, ischemia and inflammation. Acta Physiol Scand Suppl. 1986;548:9-37.
14. Gammper TJ, Zhang F, Mofakhami NF, Morgan RF, Amiss R, Hoard MA, et al. Beneficial effect hyperbaric oxygen on island flaps subjected to secondary venous ischemia. Microsurgery. 2002;22(2):49-52.
15. Zhang F, Oswald T, Lin S, Cai Z, Lei M, Jones M, et al. Vascular endothelial growth factor (VEGF) expression and effect of exogenous VEGF on survival of random flap in the rat. Br J Plast Surg. 2003;56(7):653-9.
16. Moreschi D Jr, Fagundes DJ, Hernandes L, Haapalainen EF. Effects of prostaglandin E(1) in the genesis of blood capillaries in the ischemic skeletal muscle of rats: ultrastructural analysis. Ann Vasc Surg. 2008;22(1):121-6.
17. Ito WD, Arras M, Scholz D, Winkeler B, Htun P, Schaper W. Angiogenesis but not collateral growth is associated with ischemia after femoral artery occlusion. Am J Physiol. 1997;273(3 Pt 2):H1255-65.
18. Zheng Y, Yi C, Xia W, Ding T, Zhou Z, Han Y, et al. Mesenchymal stem cells transduced by vascular endothelial growth factor gene for ischemic random skin flaps. Plast Reconstr Surg. 2008;121(1):59-69.
19. Korn C, Scholz SR, Gimadutdinow O, Pingoud A, Meiss G. Involvement of conserved histidine, lysine and tyrosine residues in the mechanism of DNA cleavage by the caspase-3 activated DNase CAD. Nucleic Acids Res. 2002;30(6):1325-32.
20. Erdmann D, Pippen AM, Moquin KJ, Sweis R, Niklason LE, Levin LS, et al. Immunohistochemical identification of vascular endothelial growth factor in pig latissimus dorsi musculocutaneous flaps following ischemia-reperfusion injury. Ann Plast Surg. 2004;53(4):398-403.
1. Plastic surgeon, associate member of the Sociedade Brasileira de Cirurgia Plástica (SBCP), doctor, professor at the Department of Surgery of the Universidade Católica de Pelotas (Catholic University of Pelotas), Pelotas, RS, Brazil
2. Doctor, associate professor of Operative Technique and Experimental Surgery at the Department of Surgery of the Universidade Federal de São Paulo (Federal University of São Paulo), São Paulo, SP, Brazil
3. Student of Medicine at the Universidade Federal de Pelotas (Federal University of Pelotas), President of the Liga Acadêmica de Cirurgia Plástica of the Federal University of Pelotas, Pelotas, RS, Brazil
4. Student of Medicine at the Universidade Federal de Pelotas (Federal University of Pelotas), Pelotas, RS, Brazil
Correspondence to:
Fernando Passos da Rocha
Praça Piratinino Almeida, 13 - Centro
Pelotas, RS, Brazil - CEP 96015-290
E-mail: fprocha.sul@terra.com.br
Submitted to SGP (Sistema de Gestão de Publicações/Manager Publications System) of RBCP (Revista Brasileira de Cirurgia Plástica/Brazilian Journal of Plastic Surgery).
Article received: November 24, 2012
Article accepted: March 19, 2013
This study was performed at the Universidade Federal de São Paulo (Federal University of São Paulo), São Paulo, SP, Brazil.